Deoxychlorination
Mechanism + Description
Synthesis of Carbon-Chlorine bonds through the activation of oxygen and then SN2 or SN1 is much more commonly used than the simple chloride anion displacement of leaving groups.
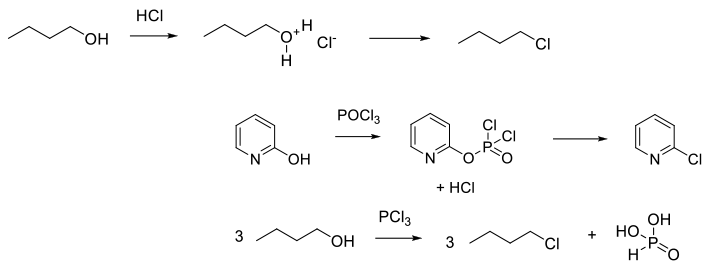
General comments
Common reagents for deoxychlorination are SOCl2, PCl3,PCl5, POCl3, PhPO(Cl)2 and HCl. These reagents are used for the conversion of C-OH or C=O bonds to chlorides – normally acid chlorides, alkyl chlorides and heteroaromatic chlorides. Other less common reagents are Ph3PCl2 and related P(V) reagents, which are usually made in situ from Ph3P and Cl2, CCl4 or C2Cl6; this is the Appel reagent. Other common regents for acid chloride formation are oxalyl chloride, phosgene, and triphosgene. In combination with Ph33P, these generate the Appel reagent. Tosyl and mesyl chlorides can convert alcohols (usually primary) to chlorides via formation of the sulphonate ester, followed by SN2 displacement by chloride. HCl is the most atom-efficient, but least reactive reagent. The reactivity of HCl can be increased by working under pressure at higher temperatures.
Reaction between deoxychlorination regents and certain amides/formamides produces chloroiminium ions that are used as electrophilic reagents/ chlorinating reagents. Catalytic amounts of DMF added to deoxychlorination reagents generate the reagent ‘in situ.’
Despite the simple overall bond transformation, reactions with deoxychlorinating agents like POCl3 and PCl3 can proceed via complex intermediates that are hydrolytically unstable and difficult to see by conventional quenching and LC/LC-MS. Techniques like 31P NMR, in situ IR, and Raman spectroscopy are required.
Key references
Relevant scale up example
Org. Process Res. Dev. 2001, 5, 426-433.
Experimental
200 g scale
Org. Process Res. Dev. 2003, 7, 309-312.
Experimental
11 g scale
Org. Process Res. Dev. 2002, 6, 938−942.
Experimental
1 kg scale
Org. Process Res. Dev. 2004, 8, 962-963.
Experimental
6 g scale
Org. Process Res. Dev. 2011, 15, 343–352.
Experimental
42 kg scale
Org. Process Res. Dev. 2011, 15, 788–796.
Experimental
25 kg scale
Org. Process Res. Dev. 2011, 15, 1348–1357.
Experimental
38 kg scale
Org. Process Res. Dev. 2011, 15, 1348–1357.
Experimental
6 kg scale
Org. Process Res. Dev. 2013, 17, 1561−1567.
Experimental
7 kg scale
Org. Process Res. Dev. 2011, 15, 1328–1335.
Experimental
10 kg scale
Org. Process Res. Dev. 2012, 16, 156−159.
Experimental
10 kg scale
Green Review
-
Atom efficiency (by-products Mwt)
Optimal with HCl, SOCl2 and PCl3; other reagents are less atom-economical. The use of Ph3P and related P reagents is very atom inefficient and should be avoided if possible. Deoxychlorination reagents are often used in molar excess (as solvent), and reactions should be optimized to use stoichiometric amounts of reagent if at all possible. - Safety Concerns
Deoxychlorination reactions can be very exothermic. These reagents are acidic and will generate HCl, so materials compatibility needs to be considered. Most deoxychlorination reagents will react violently with water/alcohols to generate HCl. Aqueous quenching of reactions using excess PCl3, SOCl2 and POCl3 can be subject to delayed exotherm due to inhomogeneity and poor mixing. The eventual reaction produces a lot of heat and acidic gas. Deoxychlorination reagents may show incompatibility with certain solvents.
"For incident quenching POCl3": UK Chemical Reaction Hazards Forum. http://www.crhf.org.uk/incident97.html (accessed May 17, 2017).
Achmatowicz, M. M.; Thiel, O. R.; Colyer, J. T.; Hu, J.; Elipe, M. V. S.; Tomaskevitch, J.; Tedrow, J. S.; Larsen, R. D. Hydrolysis of Phosphoryl Trichloride (POCl3): Characterization, in Situ Detection, and Safe Quenching of Energetic Metastable Intermediates. Org. Process. Res. Dev. 2010, 14(16), 1490-1500. - Toxicity and environmental/aquatic impact
Most reagents are too reactive to hydrolysis to be persistent and toxic per se. High local acidity might be an issue, but there are no real concerns with low levels of chloride anions following neutralization. High chloride content can be deleterious in fresh water systems.
Longer-term environmental effects will reflect organic materials associated with the reagent. Higher mol wt organic cations (quats, ionic liquids, etc.) can be inhibitory or toxic to certain aquatic life forms, so caution needs to be exercised with aqueous wastes.
Ph3P and Ph3PO are of particular environmental concern and should not be discharged into aqueous waste streams.
Inorganic P-based reagents will generate phosphate on hydrolysis, and due to concerns over eutrophication, there may be local restrictions on levels that can be discharged.
Polychlorinated organics can be persistent and bio accumulate (C2Cl6).
CCl4 is listed under the Montreal Protocol as an ozone-depleting chemical.
The use of DMF/deoxychlorination reagents can generate the carcinogenic Me2NCOCl.
NTP (National Toxicology Program). 2016. Report on Carcinogens, Fourteenth Edition. Research Triangle Park, NC: U.S. Department of Health and Human Services, Public Health Service;Profile: Dimethylcarbamoyl Chloride.http://ntp.niehs.nih.gov/go/roc14/
Stare, M.; Laniewski, K.; Westermark, A.; Sjögren, M.; Tian, W. Investigation on the Formation and Hydrolysis of N,N-Dimethylcarbamoyl Chloride (DMCC) in Vilsmeier Reactions Using GC/MS as the Analytical Detection Method. Org. Process Res. Dev. 2009, 13(5), 857–862. - Cost, availability & sustainable feedstocks
Most deoxychlorination reagents are available at scale with varying degrees of cost, but typically much cheaper than the corresponding bromine-based reagents. - Sustainable implications
No sustainability implications for Chlorine-based reagents