Mechanism + Description
The aryl halide (or other leaving group – OTf, N2+) and boronic acid
are reacted with a Pd catalyst (with or without additional ligands) in
the presence of a base. The initial step is the oxidative addition of the
aryl halide to a Pd (O) species. If Pd is charged as Pd2+, then a
prior reduction to the active form for oxidative insertion is required.
Transmetallation of the boron coupling partner then occurs, usually
via an activated boronate anion. Reductive elimination produces the
product and the active catalyst. The active Pd(O) catalyst is ligated if it is
a monomeric species, but in some cases a Pd nanoparticle
species could be involved as the active catalyst or reservoir for the active Pd.
General comments
Typically, the reagents are mixed with a Pd complex or a mixture of a simple Pd salt/complex and added ligands to generate
the active catalyst in situ. The Pd can be added as Pd2+ or PdO. Pd(OAc)2 is the most common Pd2+ salt and Pd(Ph3)4 and
Pdx(DBA)y are the most common Pd0 catalysts. Traditional ligands for the Suzuki reaction are Ph3P and (o-Tolyl)3P, although
in the past 20 years, a wide range of other ligands – principally phospines and stabilized carbenes – have been developed
to fine-tune catalytic activity and allow for reactions with traditionally difficult or unreactive coupling partners, like aryl chlorides
and very electron-rich aryl/hetreo-aryl bromides. Depending on the catalyst system and reactivity of the coupling partners,
added ligands are not always required. Bases are typically amines like Et3N or Hunig’s base, or inorganics like NaOH,
Na/K2CO3, Na/KHCO3 and KXHYPO4.
Common side reactions that can reduce yield are proto-deboronation and the homocoupling of the boronic acid. If using
arylphospine ligands and electron-rich aryl halides, an exchange of aryl groups between the phosphine and
palladium center can occur, lowering the yield and introducing extra by-products.

O’Keefe, D. F.; Dannock, M. C.; Marcuccio, S. M. Palladium Catalyzed Coupling of Halobenzenes with Arylboronic Acids: Rôle of the Triphenylphosphine Ligand. Tetrahedron Lett. 1992, 33 (44), 6679-6680.
Key references
Billingsley, K.; Buchwald, S. L. Highly Efficient Monophosphine-Based Catalyst for the Palladium-Catalyzed Suzuki−Miyaura Reaction of Heteroaryl Halides and HeteroarylBoronic Acids and Esters. J. Am. Chem. Soc. 2007, 129 (11), 3358-3366.
Slagt, V. F.; de Vries, A. H. M.; de Vries, J. G.; Kellogg, R. M. Practical Aspects of Carbon−Carbon Cross-Coupling Reactions Using Heteroarenes. Org. Process Res. Dev. 2010, 14 (1), 30-47.
Szilvási, T.; Veszprémi, T. Internal Catalytic Effect of Bulky NHC Ligands in Suzuki–Miyaura Cross-Coupling Reaction. ACS Catal. 2013, 3 (9), 1984–1991.
Selective reaction of iodo over the bromo group in Suzuki reaction: Fray, M. J.; Dickinson, R. P.; Huggins, J. P.; Occleston, N. L. A Potent, Selective Inhibitor of Matrix Metalloproteinase-3 for the Topical Treatment of Chronic Dermal Ulcers. J. Med. Chem. 2003, 46 (16), 3514-3525.
Glasspoole, B. W.; Keske, E. C.; Crudden, C. M. Stereospecific and Stereoselective Suzuki-Miyaura Cross-Coupling Reactions. New Trends in Cross-Coupling: Theory and Applications; Catalysis Series; Royal Society of Chemistry: Cambridge, U.K., 2014; 521-550.
Manjunatha, S. G.; Rangappa, P.; Sythana, S.; Babu, S. M.; Tadiparthi, K.; Gundala, C. A Simple Way of Recycling of Homogeneous Catalyst in Suzuki Reaction. Green Chemistry Letters and Reviews. 2013, 6(1), 77-87.
Denmark, S. E.; Ambrosi, A. Why You Really Should Consider Using Palladium-Catalyzed Cross-Coupling of Silanols and Silanolates. Org. Process Res. Dev. 2015, 19 (8), 982-994.
Kantchev, E. A. B.; O’Brien, C. J.; Organ, M. G. Palladium Complexes of N-Heterocyclic Carbenes as Catalysts for Cross-Coupling Reactions—A Synthetic Chemist’s Perspective. Angew. Chem., Int. Ed. 2007, 46 (16), 2768-2813.
Dreher, S. D.; Lim, S.; Sandrock, D. L.; Molander, G. A. Suzuki-Miyaura Cross-Coupling Reactions of Primary Alkyltrifluoroborates with Aryl Chlorides. J. Org. Chem. 2009, 74 (10), 3626-3631.
Slagt, V. F.; de Vries, A. H. M.; de Vries, J. G.; Kellogg, R. M. Practical Aspects of Carbon−Carbon Cross-Coupling Reactions Using Heteroarenes. Org. Process Res. Dev. 2010, 14 (1) , 30–47.
Kondolff, I.; Doucet, H.; Santelli, M. Tetraphosphine/Palladium Catalysed Suzuki Cross-Coupling Reactions of Aryl Halides with Alkylboronic Acids. Tetrahedron. 2004, 60 (17), 3813-3818.
Peh, G.; Kantchev, E. A. B.; Er, J.; Ying, J. Y. Rational Exploration of N-Heterocyclic Carbene (NHC) Palladacycle Diversity: A Highly Active and Versatile Precatalyst for Suzuki-Miyaura Coupling Reactions of Deactivated Aryl and Alkyl Substrates. Chem. Eur. J. 2010, 14 (13), 4010-4017.
Hoshi, T.; Honma, T.; Mori, A.; Konishi, M.; Sato, T.; Hagiwara, H.; Suzuki, T. An Active, General, and Long-Lived Palladium Catalyst for Cross-Couplings of Deactivated (Hetero)aryl Chlorides and Bromides with ArylboronicAcids. J. Org. Chem. 2013, 78 (22), 11513-11524.
Molander, G. A.; Trice, S. L. J.; Kennedy, S. M. Scope of the Two-Step, One-Pot Palladium-CatalyzedBorylation/Suzuki Cross-Coupling Reaction Utilizing Bis-BoronicAcid. J. Org. Chem. 2012, 77 (19), 8678-8688.
Billingsley, K. L.; Anderson, K. W.; Buchwald, S. L. A Highly Active Catalyst for Suzuki-Miyaura Cross-Coupling Reactions of HeteroarylCompounds. Angew. Chem., Int. Ed. 2006, 45 (21), 3484-3488.
Chow, W. K.; So, C. M.; Lau, C. P.; Kwong, F. Y. A General Palladium Catalyst System for Suzuki-Miyaura Coupling of Potassium Aryltrifluoroborates and Aryl Mesylates. J. Org. Chem. 2010, 75 (15), 5109-5112.
Ikawa, T.; Saito, K.; Akai, S. Palladium-Catalyzed One-Pot Cross-Coupling of Phenols Using Nonafluorobutanesulfonyl Fluoride. Synlett. 2012, 23 (15), 2241-2246.
Relevant Scale-Up Examples with Scheme

Org. Process Res. Dev. 2009, 13 (4), 751–759.
Experimental
15 kg scale
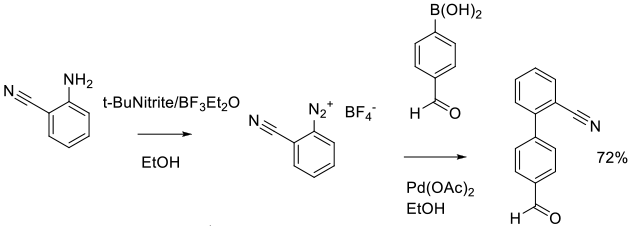
Org. Process Res. Dev. 2014, 18 (9), 1128−1136.
Experimental
500 g scale
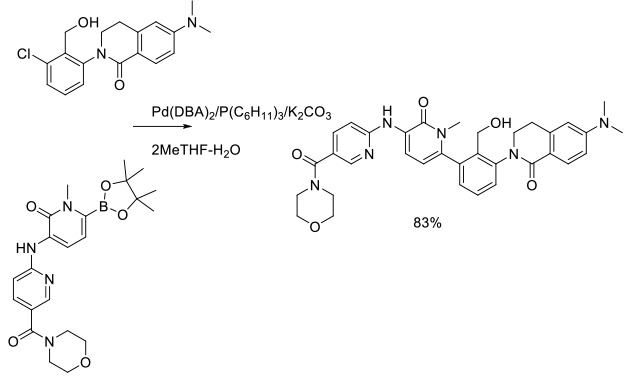
Org. Process Res. Dev. 2014, 18 (1), 228−238.
Experimental
65 g scale
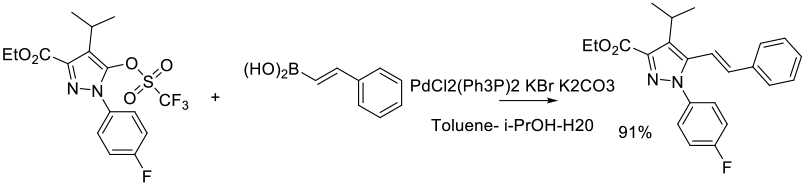
Org. Process Res. Dev. 2011, 15 (1), 148–157.
Experimental
3 kg scale
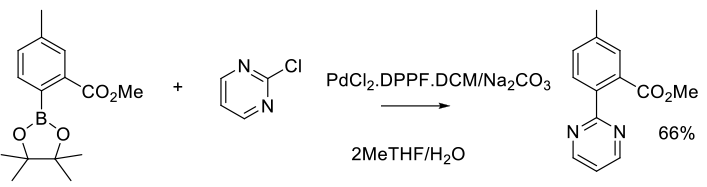
Org. Process Res. Dev. 2014, 18 (1), 228−238.
Experimental
4.5 kg scale
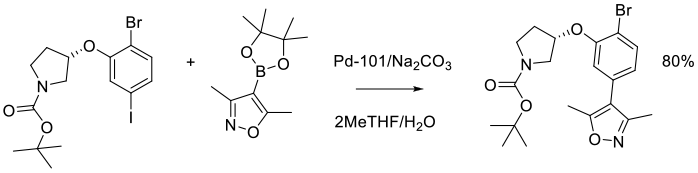
Org. Process Res. Dev. 2014, 18 (1), 228−238.
Experimental
3 kg scale

Org. Process Res. Dev. 2015, 19 (7), 721−734.
Experimental
7 kg scale
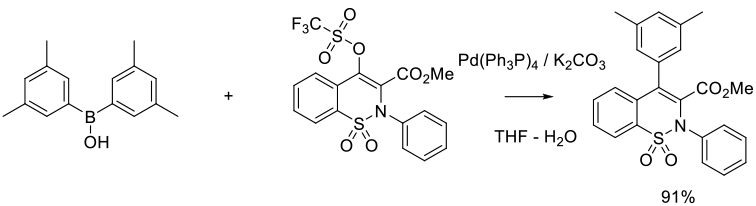
Org. Process Res. Dev. 2015, 19 (7), 721-734
Experimental
2.5 kg scale
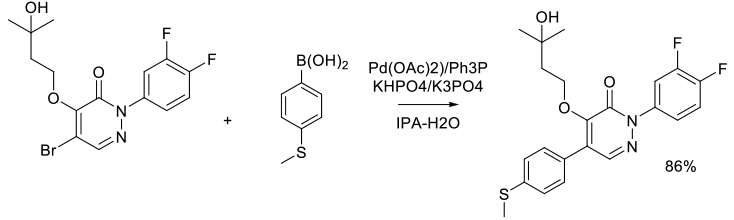
Org. Process Res. Dev. 2006, 10 (3), 512-517.
Experimental
4 kg scale
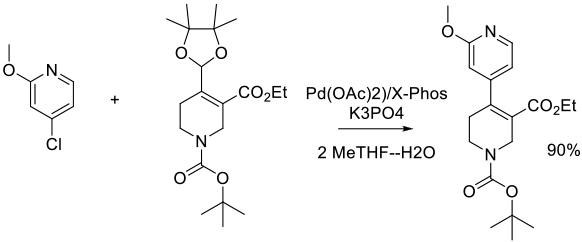
Org. Process Res. Dev. 2011, 15 (5), 1138–1148.
Experimental
2.4 kg scale
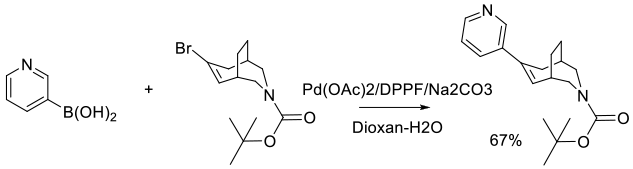
Org. Process Res. Dev. 2011, 15 (5), 1138–1148.
Experimental
1.5 kg scale

Org. Process Res. Dev. 2010, 14 (4), 849–858.
Experimental
3 kg scale