Catalytic Boron Reagents
Mechanism + Description
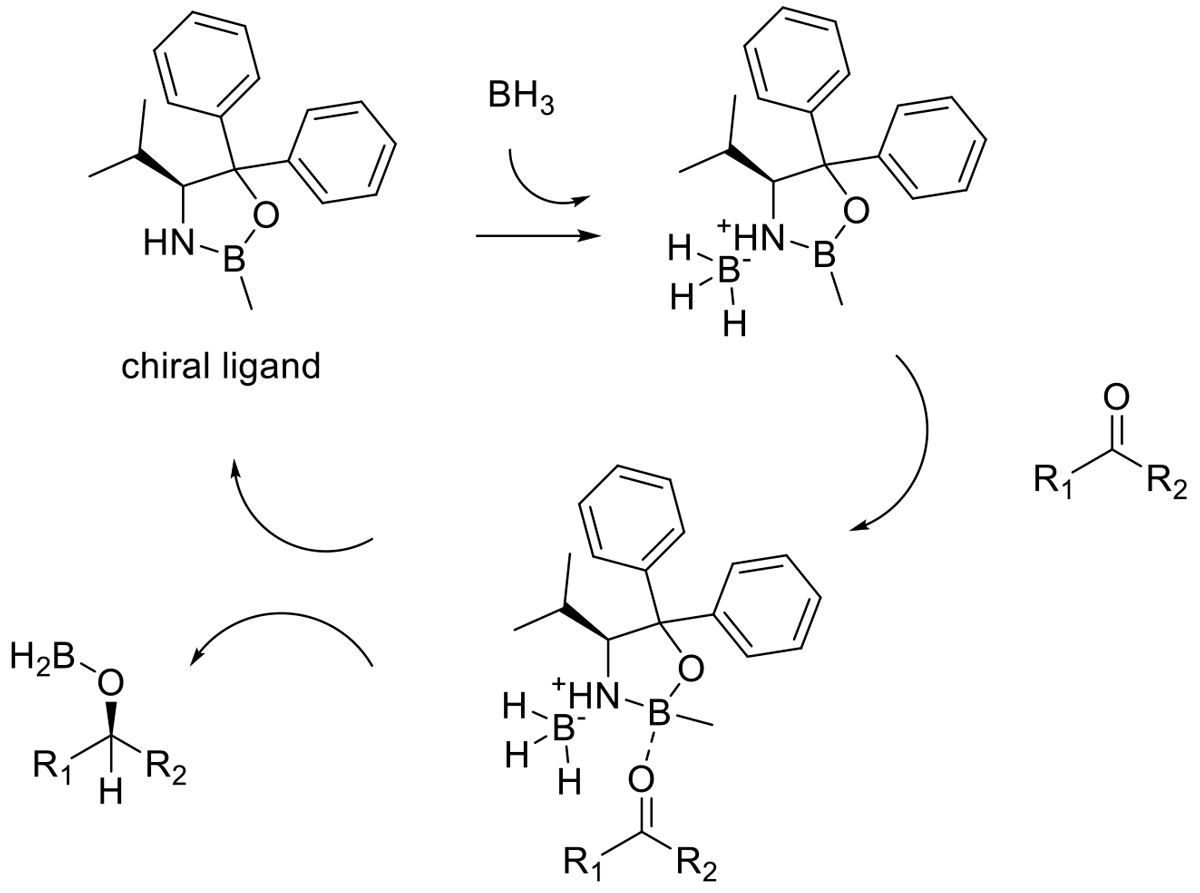
A chiral catalyst forms an active species with a reducing agent, typically a borane or aluminium hydride reagent. The ketone coordinates with a Lewis acidic site and the reducing agent is delivered to one prochiral face of the ketone. The chiral catalyst can then recycle, and the product alcohol is released on hydrolysis.
General comments
A chiral catalyst, either preformed, or synthesised in situ, is used to reduce the ketone using an added hydride source. The catalyst consists of chiral ligand(s) and an electrophilic group, usually a boron species. An added ketone is typically activated by coordination to the Lewis acidic site, and the hydride source is coordinated to a Lewis basic site. Hydride is then transferred to one prochiral face of the ketone.
BH3 complexes are often used as the hydride source. Of course, in order to gain high ee in the product alcohol, reduction by the catalytic species must be much faster than any uncatalyzed background reduction by the added hydride source. This can be achieved by correct choice of catalyst/hydride source combination, temperature, and slow addition of the hydride source to the reaction mixture avoiding a large excess of the uncomplexed hydride source.
Key references
Relevant scale up examples
Org. Proc. Res. Dev. 2009, 13, 933–935.
15 g scale
Org. Proc. Res. Dev. 2012, 16, 710−713.
6 kg scale
Org. Proc. Res. Dev. 2011, 15, 1247–1255.
2 g scale
Org. Proc. Res. Dev. 2003, 7, 285-288.
2 kg scale
Green Review
-
Atom efficiency (by-products, molecular weight)
Reduction with stoichiometric boron reagents has very poor atom economy. Addition of two hydrogens to the substrates generates by-products of 354 molecular weight. Generally such reactions also consume very large volumes of solvent in removing these by-products and are thus very mass inefficient.
Reduction with catalytic boron complexes are much better, however the catalyst stoichiometry needs to be optimized.
With optimized metal and ligand stoichiometry, catalytic metals and ligands have negligible contribution to the atom/mass intensity. Hydrogen is typically used in large molar excess, but this is non-polluting.
Biocatalysis is an efficient catalytic technology, but enzyme loading should be optimized and steps using stoichiometric reagents, like cofactor recycle, should be optimized to avoid unnecessary excess of reagents. - Safety Concerns
No major concerns around scaling asymmetric hydrogenation reactions beyond those for handling hydrogen at pressure. Generally, the lowest pressure for acceptable performance is recommended for safety, but this can be at the expense of the S/C ratio—many catalysts performing better ( TON & TOF) at higher H2 pressure (although a few give better ee at lower H2 pressure). Exotherms maybe experienced when hydrogen is introduced to the reactor.
No real concerns—enzymes are non-toxic and readily biodegradable. If live GMO cells are use, these need to be passivated before discharge into the environment. - Toxicity and environmental/aquatic impact
Main concern is around loss of precious metal/ heavy metal catalysts into waste streams. Most precious and heavy metal levels are tightly regulated. The same applies to potential metal carry through into the API. See later slide for more details on permitted. Hydrophobic, high mol. weight phosphines and phosphine oxides can be persistent and bioaccumulative and should not be discharged into aqueous waste streams.
Relevant regulatory guidance on permitted metal levels in API’s should be consulted.
For biocatalysis, there are no real concerns—enzymes are non-toxic and readily biodegradable. If viable GMO cells are use, these need to be passivated before discharge into the environment.
There may be issues with discharging aqueous waste with high boron content. Emerging data suggest boron compounds maybe more ecotoxic then previously thought. The compounds and by-products from stochiometric boron reagents are persistent and can accumulate in the environment. - Cost, availability & sustainable feedstocks
With high catalytic efficiency, this methodology can be an economical way to access chiral molecules. Precious metals like Ru, Ir and Rh are commodities and thus the price and availability can fluctuate widely with demand and general global economic cycles. Base metals are cheaper and much more abundant.
Enzymes are produced by fermentation from natural sustainable feedstocks - Sustainable implications
All metals have a high LCI impact from mining and refining operations, so use should be catalytic with efficient recovery and recycle. Ru, Ir and Rh are the most commonly used precious metal for chiral hydrogenation and these are rated at high risk of depletion. No concern for abundant base metals like Co, Ni, Fe, etc.
Enzymes are made via fermentation and cloned and recombinant enzymes would be at no risk from depletion. Enzymes break down in the environment and the constituent amino acids which are recycled in nature. The use of over expressed recombinant enzymes is generally much better than natural enzymes on a life cycle impact basis.