Metal-catalyzed Approaches to Aryl Thioethers
Mechanism + Description
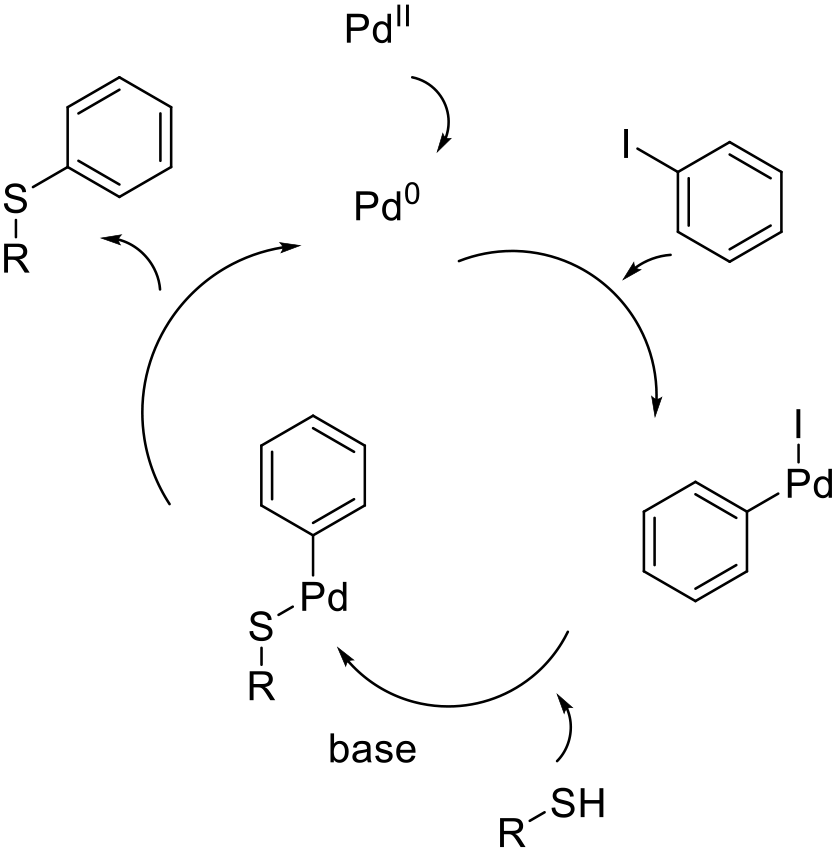
The metal catalyzed arylation of thiols follows the usual pathway of oxidative insertion of a metal catalyst into an aryl halide/sulfonate followed by coordination of the thiol followed by reductive elimination to give the product. The role of the base is to deprotonate the thiol prior to, or after, coordination to the metal catalyst.
General comments
Metal catalyzed thiolation of aryl/heteroaryl halides and sulfonates is employed when the aryl substrate is not reactive enough for a classical uncatalyzed SNAr substitution. Typically Buchwald-Hartwig conditions are used with Pd catalysis; but there is interest in Cu catalysis. The very harsh conditions traditionally used for Ullman-type coupling can be moderated by the use of appropriate ligands. Typically coupling partners are halides/sulfonates, e.g., I, Br, Cl, Triflates, mesylates, tosylates, and thiols or thiol surrogates that generate the free thiol in situ. As with all metal catalyzed couplings of this nature (C-N/O/S), the choice of ligand is crucial to the success of the reaction. Typical ligands are based on phosphines/bisphosphines; carbene ligands and nitrogen ligands for Cu bases are typically inorganics like NaOH, Na/K/Cs2CO3, Na/KHCO3 and KXHYPO4 or alkoxides – Na/K t-BuO. Occasionally organic amine bases are employed.
In common with most metal-catalyzed carbon-heteroatom bond formations, there is a drive to develop greener more sustainable catalytic reactions for thioether synthesis. These include substituting Pt group metals like Pd or base metals like Cu, Co, Ni, Fe, and avoiding halogens and organohalogen intermediates by synthetic approaches like C-H activation, decarboxylative coupling and redox neutral ‘borrowing hydrogen’ strategies.
Key references
Relevant scale up examples
Org. Process Res. Dev. 2010, 14, 346–350.
400 g scale
Org. Process Res. Dev. 2008, 12, 869–876
45 kg scale
Org. Process Res. Dev. 2011, 15, 1046–1051
30 kg scale
Org. Process Res. Dev. 2014, 18, 266−274.
140 kg scale
Org. Process Res. Dev. 2015, 19, 1944–1953.
30 kg scale
Green Review
-
Atom efficiency (by-products, molecular weight)
With optimized metal and ligand stoichiometry, catalytic metals and ligands have negligible contribution to the atom/mass intensity. By-products are inorganic salts/amine salts/alcohols. In terms of leaving groups Cl<Br<Mesylate<I<triflate<tosylate. - Safety Concerns
No major concerns around scaling metal-catalyzed thioether formation reactions. Lower mol. wt. alkylphosphines can be highly flammable. H2S or volatile low mol. wt. alkyl thiols like MeSH are highly toxic. All metals will have safety limits in final API product. - Toxicity and environmental/aquatic impact
Main concern is around loss of precious metal/ heavy metal catalysts into waste streams. Most precious and heavy metal levels are tightly regulated. The same applies to potential carry through into the API. Some Ni salts are sensitizers and carcinogens and listed on the EU SVHC list. Hydrophobic, high mol. wt. phosphines and other ligands by-products like phosphine oxides and can be persistent and bioaccumulative and should not be discharged into aqueous waste streams.
Simple bases like Na/K hydroxides, carbonates, bicarbonates and phosphate are preferred over organic amines and alkoxides. Local regulations may limit concentrations of phosphate that can be discharged in aqueous waste. Lower mol. wt. alkylsulfides, thioethers and H 2S are highly malodorous and reaction off-gases should be scrubbed with an oxidant like NaOCl. - Cost, availability & sustainable feedstocks
With high catalytic efficiency, this methodology can be an economical way to access aryl/heteroaryl amine compounds. All metal catalysts have a high LCI from mining and refining and would need to be efficiently recovered and recycled for precious metals. - Sustainable implications
All metals have a high LCA impact from mining and refining operations, so use should be catalytic with efficient recovery and recycle. Pd is currently the most commonly used precious metal for hydrogenation and this is rated at high risk of depletion. No concern for abundant base metals like Ni, Cu, Fe, etc. If aryl iodides are used, incineration of waste streams could be problematic (iodine content). Limited utility for waste by-products. Iodine is an element at medium to high risk of depletion. High LCA reagent, although it is possible to recover iodide from waste materials. Li and Cs bases should be avoided if possible.
Updated ICH Guidelines for Metals in APIs
ICH q3d guideline for elemental impurities 2018
When using metal catalysts, it is important to check that any wastes discharged to the environment have levels of precious/heavy metals less than levels permitted by local legislation.
There are also strict limits for metals in API/finished medicines to ensure patient safety.
For guidance, see limits in µg gram-1 and permitted daily exposures