Cyclopropanation via Metal Carbenes/Carbenoids – Including Chiral Transfer
Mechanism + Description
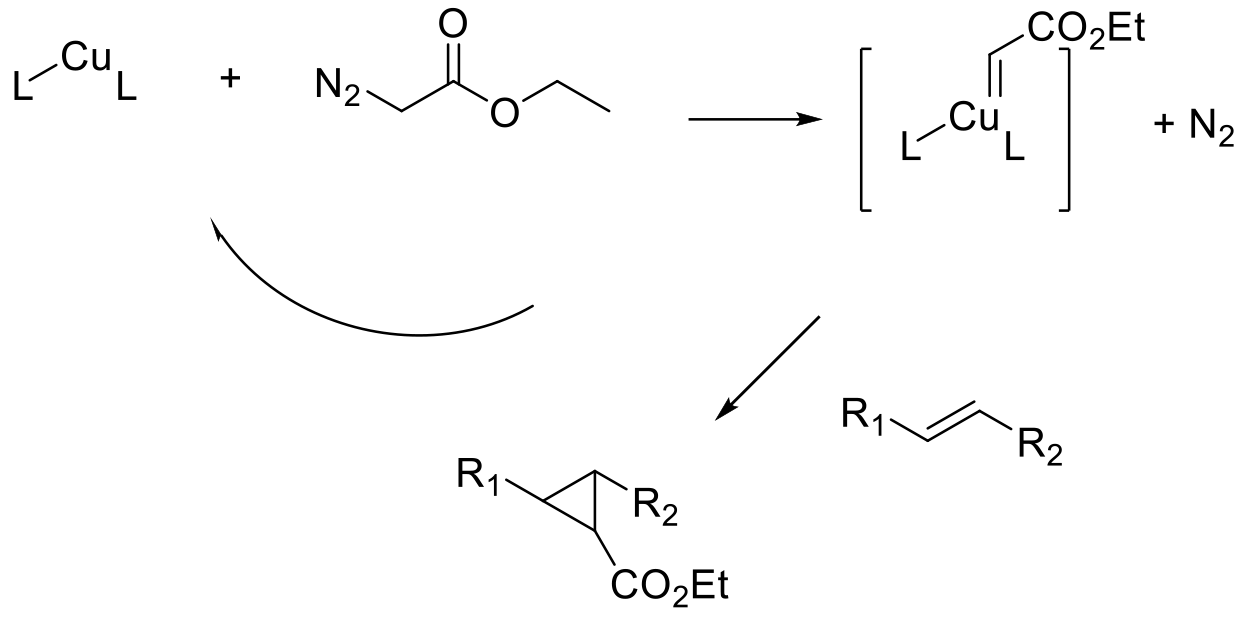
Carbene precursors are decomposed, or carbenes formed, in the presence of metals. The metal carbenoid species are generally much more stable and less reactive than free carbenes and undertake synthetically useful reactions like the transfer of the carbene fragment to an alkene, generating the cyclopropane ring.
General comments
The generation and subsequent reaction of stable metal carbenoids to generate cyclopropanes is probably the most widely used methodology to generate cyclopropanes. In the presence of chiral additives/ligands, the transfer of the carbene can be done in an enantioselective fashion to prepare chiral cyclopropanes. The Simmons-Smith cyclopropanation was probably the first widely adopted methodology. This transformation utilizes a stoichiometric Zn carbenoid generated from Zn metal or alloys, or Et2Zn and diiodomethane generating in situ ZnI(CH2I), EtZnCH2I, or Zn(CH2I)2 as the active carbenoid reagents.
The reaction has for the most partbeen superseded by the use of metal complexes to decompose diazoalkanes to metal carbenes and nitrogen. Electron-rich olefins are ideal substrates for cyclopropanation, and the reaction can be run in classical intermolecular fashion or in an intramolecular mode where the carbene and alkene are part of the same molecule. Many different metals have been employed for carbene transfer reactions including Rh, Ru, Ir, Au, Pt, Cu, Fe and Ni. For prochiral alkenes, chirality can be induced by using a chiral ligand. Many classes of chiral ligands have been described in the literature, C2-symmetric chiral bisoxazolines (BOX) ligands have found wide utility, but many others have been used, and several are commercially available as free ligands or preformed metal complexes.
1,1,-di-substituted and mono-substituted alkenes are preferred substrates for chiral cyclopropanation, and as a general rule of thumb, the more complex, substituted and sterically crowded the alkene is, the more difficulty one will have to get an efficient catalytic cyclopropanation with high e.e. Many chiral cyclopropanation reactions have downstream upgrading steps to improve e.e./isomer ratios. Metal carbenoid cyclopropanations can benefit from extensive screening of available catalysts to maximize the desired isomer ratios.
Key references
Relevant scale up examples
Org. Process Res. Dev. 2018, 22, 1393–1408.
20 kg scale
Org. Process Res. Dev. 2016, 20, 495−502.
gram scale
Org. Process Res. Dev. 2008, 12, 168–177.
8 kg scale
Relevant scale up examples: metal carbenoids, including chiral transfer
Org. Process Res. Dev. 2011, 15, 1192–1200.
100 g scale
Org. Process Res. Dev. 2016, 20, 786−798.
33 kg scale
Green Review
-
Atom efficiency (by-products, molecular weight)
With optimized metal and ligand stoichiometry, catalytic metals and ligands have negligible contribution to the atom/mass intensity. Stoichiometric reactions such as Simmons-Smith use a lot of Zn and high mol. wt. organoiodides, and Kulinkovich cyclopropanation uses stoichiometric Ti reagents. - Safety Concerns
Organozinc derivatives can be highly flammable. Diazoalkanes like diazomethane, aryl, alkenyl, and alkynyldiazo compounds are potentially explosive and appropriate care taken when scaling up such reactions. All metals have limits in final API product – see later. - Toxicity and environmental/aquatic impact
The main concern is around loss of precious metal/ heavy metal catalysts into waste streams. Most precious and heavy metal levels are tightly regulated. The same applies to potential metal carry through into the API. See later for more details on permitted levels. Hydrophobic, high mol. wt. phosphines and phosphine oxides (ligands) can be persistent and bioaccumulative and should not be discharged into aqueous waste streams.
Waste streams containing iodide and organoiodide residues can be expensive to dispose of in an environmentally responsible manner. Incineration of waste streams could be problematic due to iodine content. - Cost, availability & sustainable feedstocks
With high catalytic efficiency, this methodology can be an economical way to access chiral molecules. Precious metals such as like Ru/Ir/Rh are commodities and thus the price and availability can fluctuate widely with demand and general global economic cycles. Since 2010, the price of Rh has dropped from ~$2500 to ~ $800 per oz. In 2007, Rh approached $10,000 per oz. - Sustainable implications
All metals have a high LCI impact from mining and refining operations, so use should be catalytic with efficient recovery and recycle. Ru/Ir/Rh are the most commonly used precious metal for chiral hydrogenation and these are rated at high risk of depletion. No concern for abundant base metals like Co, Ni, Fe. etc. Zn is at serious threat of depletion in next 100 years.
Iodine is an element at medium to high risk of depletion. Ti is abundant.
Updated ICH Guidelines for Metals in API’s
ICH q3d guideline for elemental impurities 2018
When using metal catalysts, it is important to check that any wastes discharged to the environment have levels of precious/heavy metals less than levels permitted by local legislation. There are also strict limits for metals in API/finished medicines to ensure patient safety. Please check the ICH q3d guideline for elemental impurities (reference below).
Regulatory guidance and requirements
For information on impurities in pharmaceuticals, e.g., residual solvents, metal levels, genotoxic impurities, please check current information on the following sites:
The International Council for Harmonisation of Technical Requirements for Pharmaceuticals for Human Use (ICH)
U.S. Food and Drug Administration (FDA)
European Medicines Agency (EMEA)
For current legislation on chemicals/reagents, human health or environmental issues, please check current information on the following sites:
European Chemicals Agency (ECHA)
U.S. Environmental Protection Agency (EPA)
Environmental laws/regulations are regional, time-specific, and subject to change.