Transaminase
Mechanism + Description
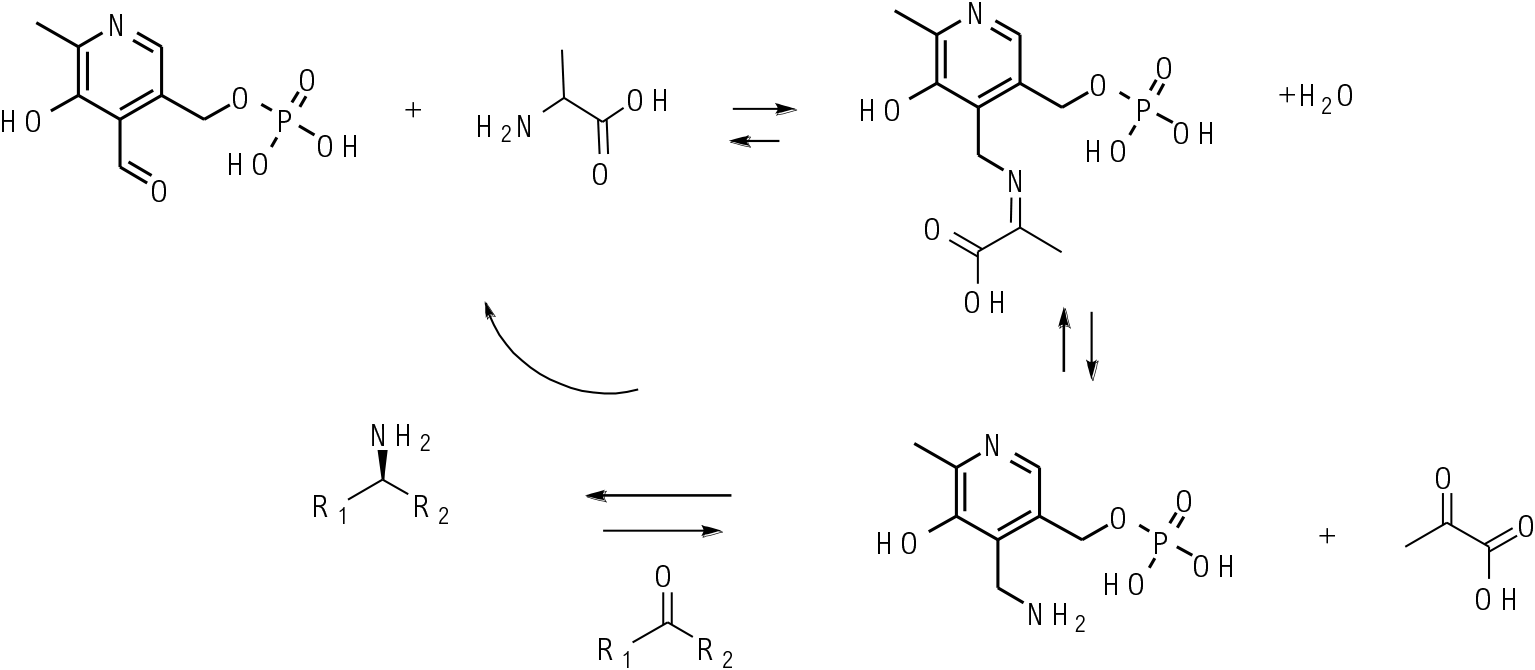
Transaminases work through the cofactor pyridoxal phosphate. This is converted to pyridoxal amine in the enzyme active site and this reacts with a ketone in essentially a reverse process to produce a chiral amine product and regenerate the pyridoxal phosphate cofactor. The chiral environment of the enzyme active site delivers the amine to one prochiral face of the ketone substrate.
General comments
Transaminases are used to make chiral amines from ketones or amino acids from α keto acids. An amine donor is used to supply the NH3 equivalent. This produces a ketone/ keto acid as a by-product. Thus, in transaminase reactions, there is little thermodynamic drive to push the reaction towards the products and various ways need to be employed to drive the equilibrium in the desired direction. A range of amine donors can be employed such as amino acids and organic amines such as isopropylamine, methylbenzylamine or other amines in some cases. The equilibrium is displaced by enzymically or physically removing the keto or keto acid by-product from the amine donor, thus pulling the equilibrium in the desired direction. As well as synthesis mode, transaminases can be used for the kinetic resolution of racemic amine mixtures using a simple NH3 acceptor such as pyruvate to convert one amine enantiomer to the corresponding ketone.
Key references
Relevant scale up examples
Science 2010, 239, 305−309
multi kg scale
Org. Process Res. Dev. 2012, 16, 1953−1966
30 g scale
Org. Process Res. Dev. 2017, 21,871−877
25 kg scale
Green Review
-
Atom efficiency (by-products, molecular weight)
Catalytic technology—but enzyme loading should be optimized and steps using stoichiometric reagents like cofactor recycle should be optimized to avoid unnecessary excess of reagents. - Safety Concerns
Generally considered a safe technology to scale-up. Issues—enzymes/proteins can be sensitizers by inhalation, and some by mode of action can be skin irritants (proteases). If viable GMO cells are used, local regulations relating to use of GMOs need to be followed. - Toxicity and environmental/aquatic impact
No real concerns—enzymes are non-toxic and readily biodegradable. If viable GMO cells are used, these need to be passivated before discharge into the environment. - Cost, availability & sustainable feedstocks
Enzymes are produced from natural sustainable feedstocks. - Sustainable implications
Enzymes are made via fermentation; cloned and recombinant enzymes would be at no risk from depletion. Enzymes break down in the environment and the constituent amino acids are recycled in nature. The use of over expressed recombinant enzymes is generally much better than natural enzymes on a life cycle impact basis.